by Thea Singer
Honeybees have been dying in record numbers, threatening the continued production of nutritious foods such as apples, nuts, blueberries, broccoli, and onions. Without bees to pollinate these crops, the environmental ecosystem—and our health—stands in the balance. Have we reached the tipping point, where the plant-pollinator system is due to collapse?
There was no way to calculate that—until now.
Using statistical physics, Northeastern network scientist Albert-László Barabási and his colleagues Jianxi Gao and Baruch Barzel have developed a tool to identify that tipping point—for everything from ecological systems such as bees and plants to technological systems such as power grids. It opens the door to planning and implementing preventive measures before it’s too late, as well as preparing for recovery after a disaster.
The tool, described in a new paper published on Wednesday in the prestigious journal Nature, fills a longstanding gap in scientists’ understanding of what determines “resilience”—that is, a system’s ability to adjust to disturbances, both internal and external, in order to remain functional.
“The failure of a system can lead to serious consequences, whether to the environment, economy, human health, or technology,” said Barabási, Robert Gray Dodge Professor of Network Science and University Distinguished Professor in the Department of Physics. “But there was no theory that considered the complexity of the networks underlying those systems—that is, their many parameters and components. That made it very difficult, if not impossible, to predict the systems’ resilience in the face of disturbances to those parameters and components.”
“Our tool, for the first time, enables those predictions,” said Barabási, who is also a leader in Northeastern’s Network Science Institute.
Taking a system’s temperature
Barzel, a postdoctoral fellow in Barabasi’s lab who collaborated on the research and is now at Bar-Ilan University, draws an elegant analogy between the role of temperature in identifying that tipping point in a pot of water and the single parameter—a temperature equivalent, as it were—that their tool can uncover to identify the tipping point in any complex system.
As the water heats up, those parameters and components continually change. Measuring those multitudinous changes over time—a microscopic approach to assessing the water’s state—would be impossible. How, then, are we to know when the water is reaching the threshold that divides the desirable (liquid) state from the undesirable (vapor) state?
Simple: Using a single parameter—temperature. As the water in the pot reaches, say, 99 degrees Celsius, alarms go off and we know to remove it from the heat.
“Statistical physics has found that you can crunch down all of these millions of parameters and components into one number—the temperature,” said Barzel. “We take it for granted now, but that was a tremendous scientific achievement.”
The researchers’ tool similarly crunches down all the parameters and components of any complex system into a single crucial number. It enables us, essentially, to take the system’s “temperature” to determine its health and respond accordingly.
From theory to application
Of course, the interaction between, say, bees and plants in an ecological system is not the same as the interaction between water molecules in a pot, noted Barzel. “But the way of thought and the mathematical tools that we use—statistical physics—are very similar.”
A big difference, however, is that we know how to prevent the water system from collapsing: Turn off the heat before the temperature reaches 100 degrees Celsius. Indeed, the water system on its own provides a visible clue as it approaches the tipping point: bubbles. You can’t say the same for the dying bees. The problem leads naturally to the researchers’ next steps: Using statistical physics both to detect trouble in a system early on and to bring about its recovery if it has crossed the threshold.
“Once you identify the relevant parameter that controls the system’s resilience, you can begin to tackle how to manipulate that resilience—how to enhance resilience or restore resilience,” said Gao. “These are not easy questions, but our theory, by giving us a picture of the entire system, paves the way to the answers.”
Originally published in news@Northeastern on February 17, 2016.
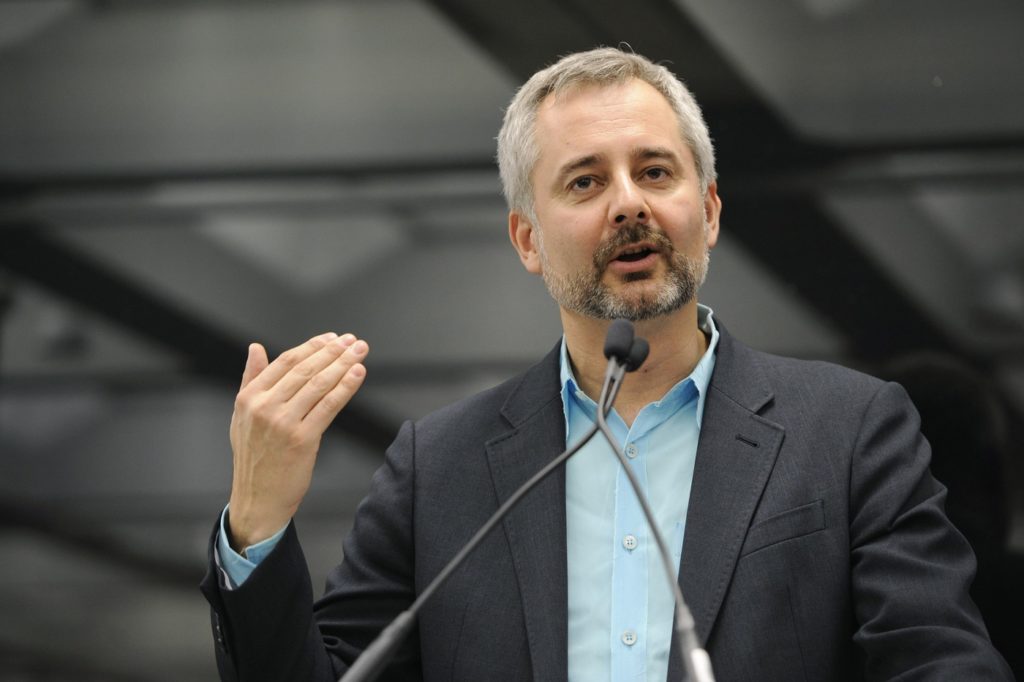
Professor of network science Albert László Barabásiphoto by Matthew Modoono/Northeastern University